Life Cycle Assessment: the scientific foundation of the Environmental Footprint methods
Life Cycle Assessment (LCA), standardised with ISO 14040/44, is a powerful and essential sustainability tool that evaluates the environmental impacts of a product or service across its entire life cycle, from raw material extraction to the end of life.
As the basis of the Product Environmental Footprint (PEF) and Organisation Environmental Footprint (OEF) methods, Life Cycle Assessment (LCA) provides a holistic approach and a framework for decision-making, enabling the comprehensive assessment and management of the environmental impacts of products and organisations. In this way, companies can better understand and reduce their environmental impacts, and use this information to inform consumers about the sustainability of their products or organisations.
Learn more about the Life Cycle Assessment (LCA) here.
Environmental Impact Categories
Life Cycle Impact Assessment (LCIA) aims to evaluate the contributions of products or organizations on different environmental issues such as climate change, water consumption, land use and resource use.
A wide range of environmental issues is covered to ensure a comprehensive assessment. The Product Environmental Footprint (PEF) and Organisation Environmental Footprint (OEF) methods aim to achieve this by addressing 16 impact categories. These are periodically updated to reflect the latest scientific knowledge and best practices, also informed by discussions within Commission’s expert groups.
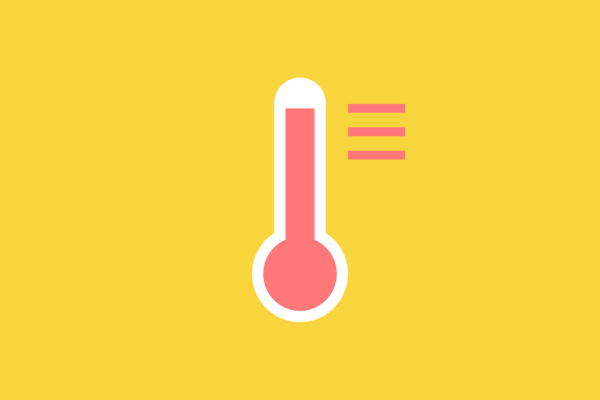
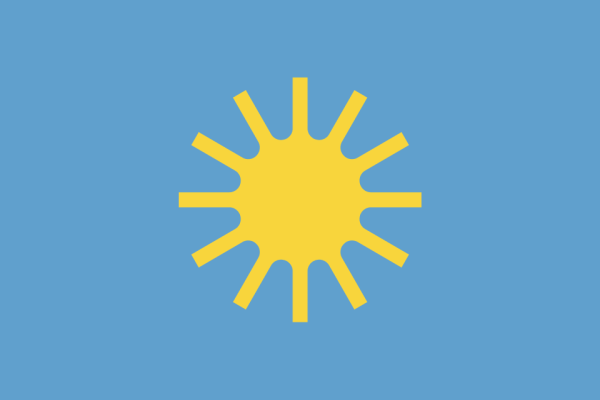

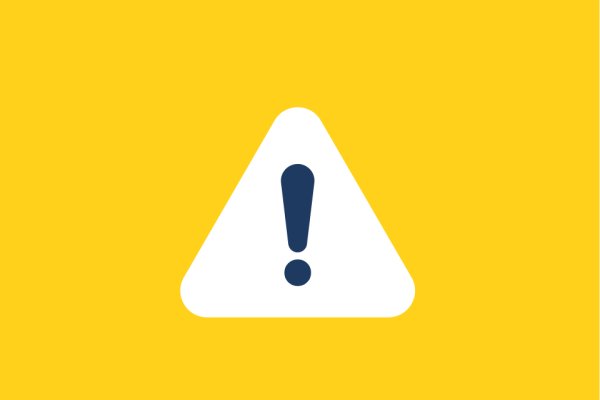
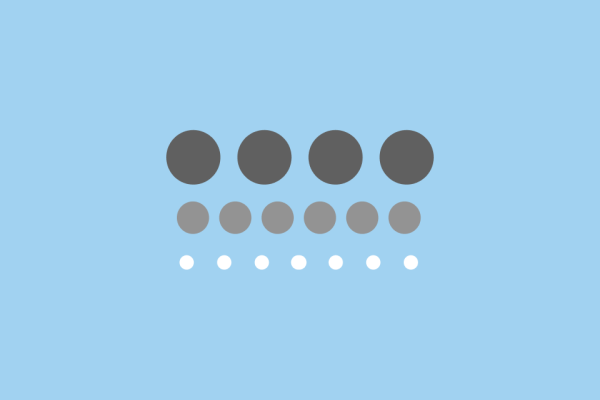

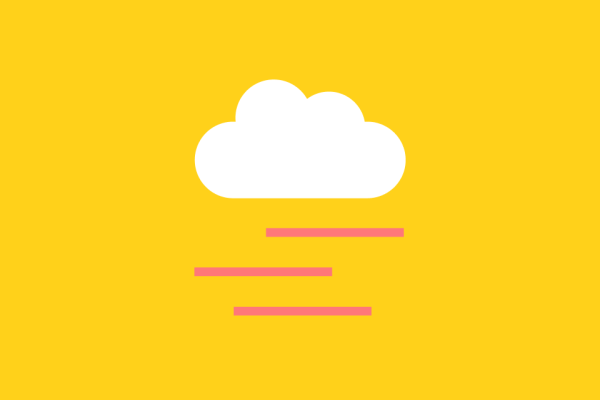

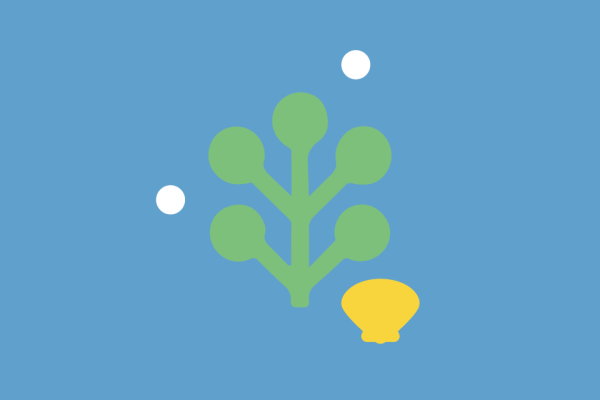
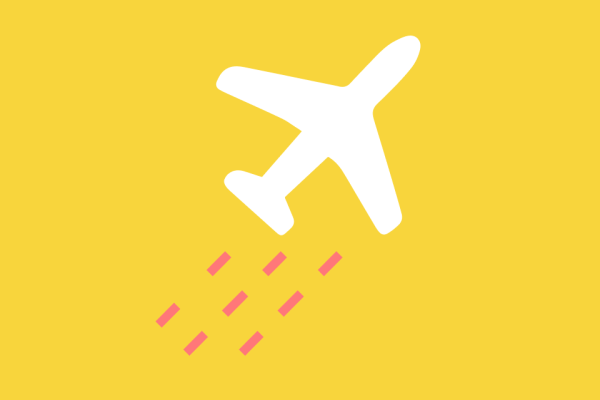

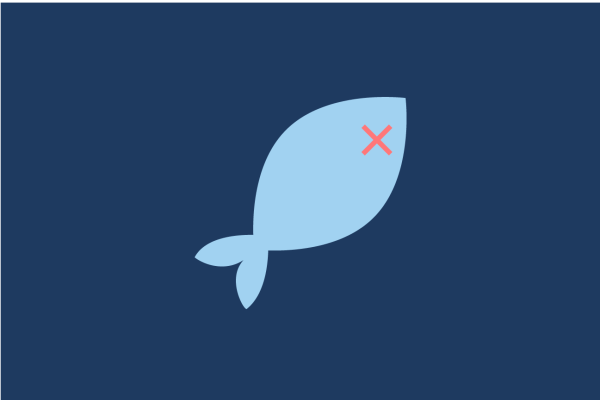
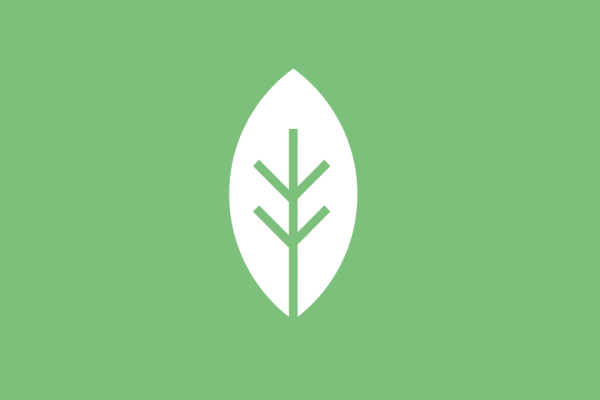

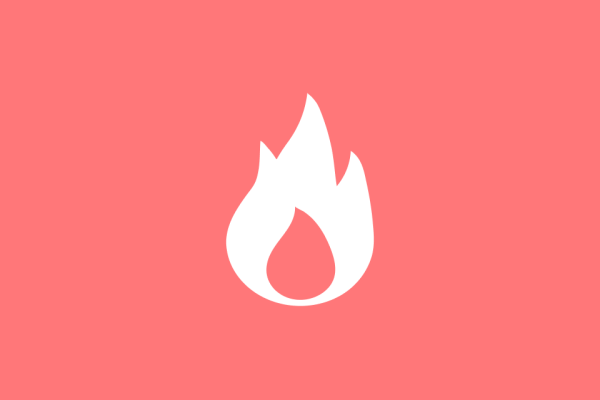
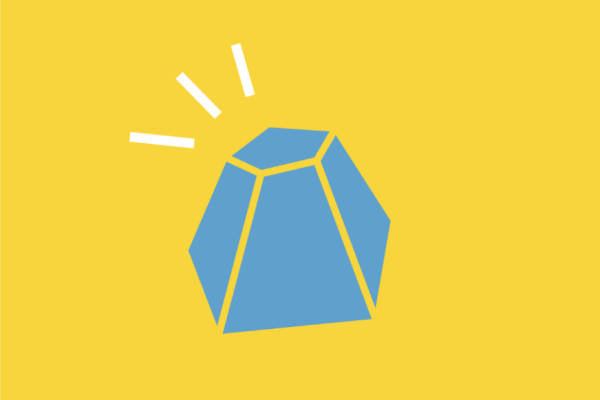
This indicator refers to the increase in the average global temperatures as result of greenhouse gas (GHG) emissions. The greatest contributor is generally the combustion of fossil fuels such as coal, oil, and natural gas. The global warming potential of all GHG emissions is measured in kilogram of carbon dioxide equivalent (kg CO2 eq), namely all GHG are compared to the amount of the global warming potential of 1 kg of CO2.
The stratospheric ozone (O3) layer protects us from hazardous ultraviolet radiation (UV-B). Its depletion increases skin cancer cases in humans and damage to plants. The potential impacts of all relevant substances for ozone depletion are converted to their equivalent of kilograms of trichlorofluoromethane (also called Freon-11 and R-11), hence the unit of measurement is in kilogram of CFC-11 equivalent (kg CFC-11 eq).
This indicator refers to potential impacts, via the environment, on human health caused by absorbing substances from the air, water and soil. Direct effects of products on human health are currently not measured. The unit of measurement is Comparative Toxic Unit for humans (CTUh). This is based on a model called USEtox.
This indicator refers to potential impacts, via the environment, on human health caused by absorbing substances from the air, water, and soil. Direct effects of products on human health are currently not measured. The unit of measurement is Comparative Toxic Unit for humans (CTUh). This is based on a model called USEtox.
This indicator measures the adverse impacts on human health caused by emissions of Particulate Matter (PM) and its precursors (e.g. NOx, SO2). Usually, the smaller the particles, the more dangerous they are, as they can go deeper into the lungs. The potential impact of is measured as the change in mortality due to PM emissions, expressed as disease incidence per kg of PM2.5 emitted.
The exposure to ionising radiation (radioactivity) can have impacts on human health. The Environmental Footprint only considers emissions under normal operating conditions (no accidents in nuclear plants are considered). The potential impact on human health of different ionising radiations is converted to the equivalent of kilobequerels of Uranium 235 (kg U235 eq).
Ozone (O3) on the ground (in the troposphere) is harmful: it attacks organic compounds in animals and plants, it increases the frequency of respiratory problems when photochemical smog (“summer smog”) is present in cities. The potential impact of substances contributing to photochemical ozone formation is converted into the equivalent of kilograms of Non-Methane Volatile Organic Compounds (e.g. alcohols, aromatics, etc.; kg NMVOC eq).
Acidification has contributed to a decline of coniferous forests and an increase in fish mortality. Acidification can be caused by emissions to the air and deposition of emissions in water and V2.0 – 25th August 2023 27 soil. The most significant sources are combustion processes in electricity, heat production, and transport. The more sulphur the fuels contain the greater their contribution to acidification. The potential impact of substances contributing to acidification is converted to the equivalent of moles of hydron (general name for a cationic form of atomic hydrogen, mol H+ eq).
Eutrophication arises when substances containing nitrogen (N) or phosphorus (P) are released to ecosystems. These nutrients cause a growth of algae or specific plants and thus limit growth in the original ecosystem. The potential impact of substances contributing to terrestrial eutrophication is converted to the equivalent of moles of nitrogen (mol N eq).
Eutrophication impacts ecosystems due to substances containing nitrogen (N) or phosphorus (P), which promotes growth of algae or specific plants. If algae grow too rapidly, it can leave water without enough oxygen for fish to survive. Nitrogen emissions into the aquatic environment are caused by fertilisers used in agriculture, but also by combustion processes. The most significant sources of phosphorus emissions are sewage treatment plants for urban and industrial effluents and leaching from agricultural land. The potential impact of substances contributing to freshwater eutrophication is converted to the equivalent of kilograms of phosphorus (kg P eq).
Eutrophication in ecosystems happens when substances containing nitrogen (N) or phosphorus (P) are released to the ecosystem. As a rule, the availability of one of these nutrients will be a limiting factor for growth in the ecosystem, and if this nutrient is added, the growth of algae or specific plants will increase. For the marine environment this will be mainly due to an increase of nitrogen (N). Nitrogen emissions are caused largely by the agricultural use of fertilisers, but also by combustion processes. The potential impact of substances contributing to marine eutrophication is converted to the equivalent of kilograms of nitrogen (kg N eq).
This indicator refers to potential toxic impacts on an ecosystem, which may damage individual species as well as the functioning of the ecosystem. Some substances tend to accumulate in living organisms. The unit of measurement is Comparative Toxic Unit for ecosystems (CTUe). This is based on a model called USEtox.
Use and transformation of land for agriculture, roads, housing, mining or other purposes. The impacts can vary and include loss of species, of the organic matter content of soil, or loss of the soil itself (erosion). This is a composite indicator measuring impacts on four soil properties (biotic production, erosion resistance, groundwater regeneration and mechanical filtration), expressed in points (Pts).
The abstraction of water from lakes, rivers or groundwater can contribute to the ‘depletion’ of available water. The impact category considers the availability or scarcity of water in the regions where the activity takes place, if this information is known. The potential impact is expressed in cubic metres (m3 ) of water use related to the local scarcity of water.
The earth contains a finite amount of non-renewable resources, such as fossil fuels like coal, oil and gas. The basic idea behind this impact category is that extracting resources today will force future generations to extract less or different resources. For example, the depletion of V2.0 – 25th August 2023 28 fossil fuels may lead to the non-availability of fossil fuels for future generations. The amount of materials contributing to resource use, fossils, are converted into MJ.
This impact category has the same underlying basic idea as the impact category resource use, fossils (namely, extracting a high concentration of resources today will force future generations to extract lower concentration or lower value resources). The amount of materials contributing to resource depletion are converted into equivalents of kilograms of antimony (kg Sb eq).